Colorado School of Mines
Colorado’s premier engineering and applied science university for 150 years and counting
Colorado School of Mines is a public R1 research university focused on applied science and engineering, producing the talent, knowledge and solutions to serve industry and benefit society – all to create a more prosperous future.
Extended Admissions Decision Deadline for First-Year Students
Accepted first-year students now have until May 15, 2024 to finalize their enrollment decision and submit a deposit.
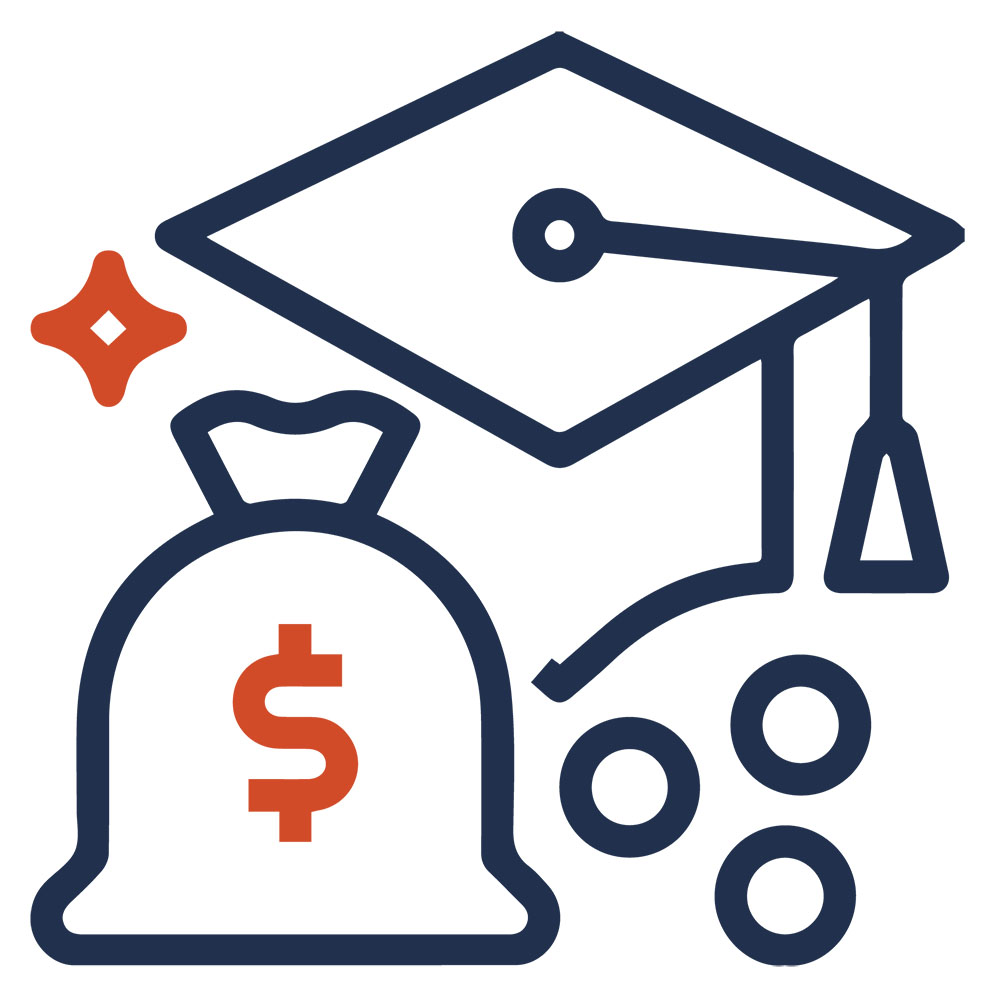
A top research university with a high ROI and recognized by Carnegie as an R1 “very high research activity” university
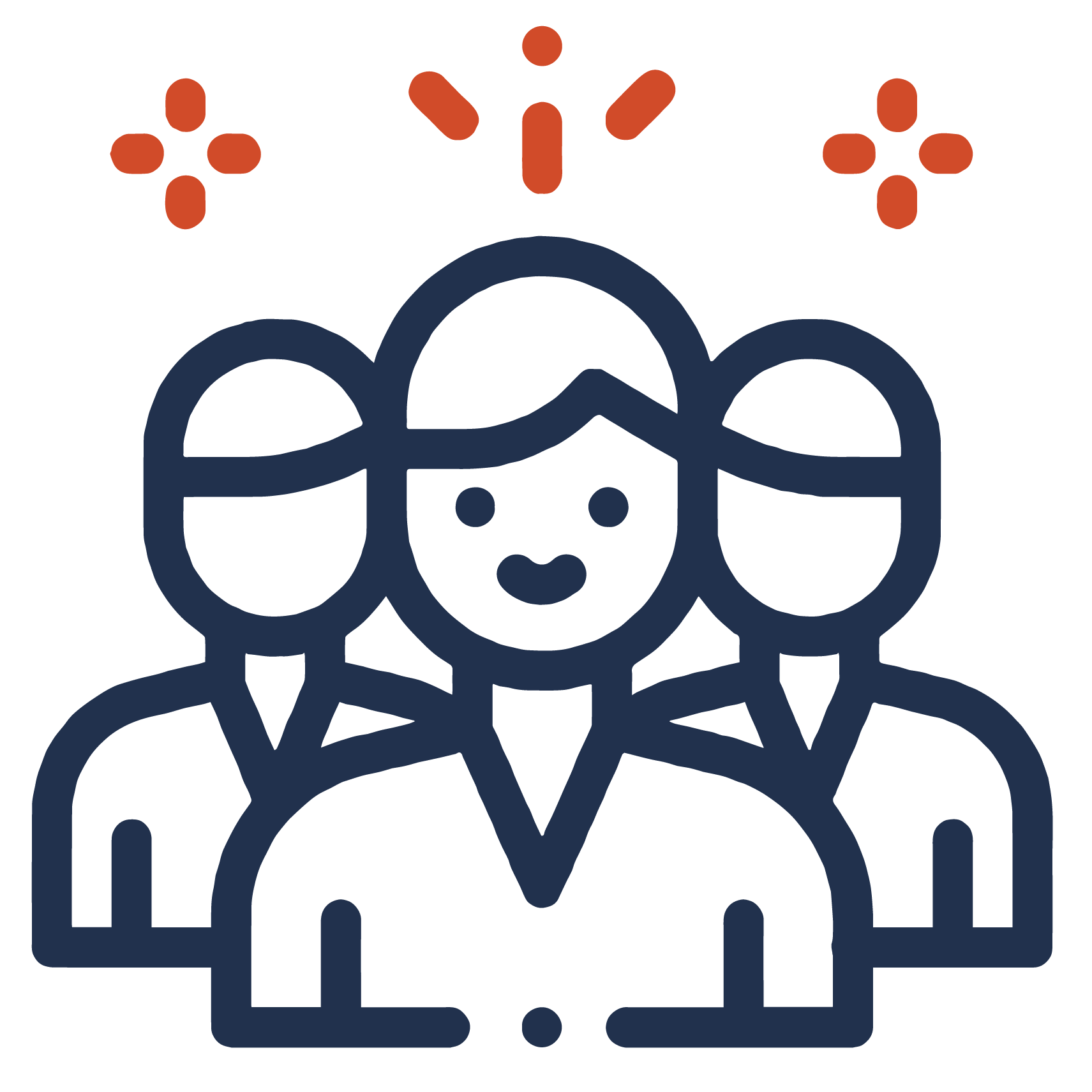
Being an Oredigger is unlike anything else. How will you make the most of your experience?
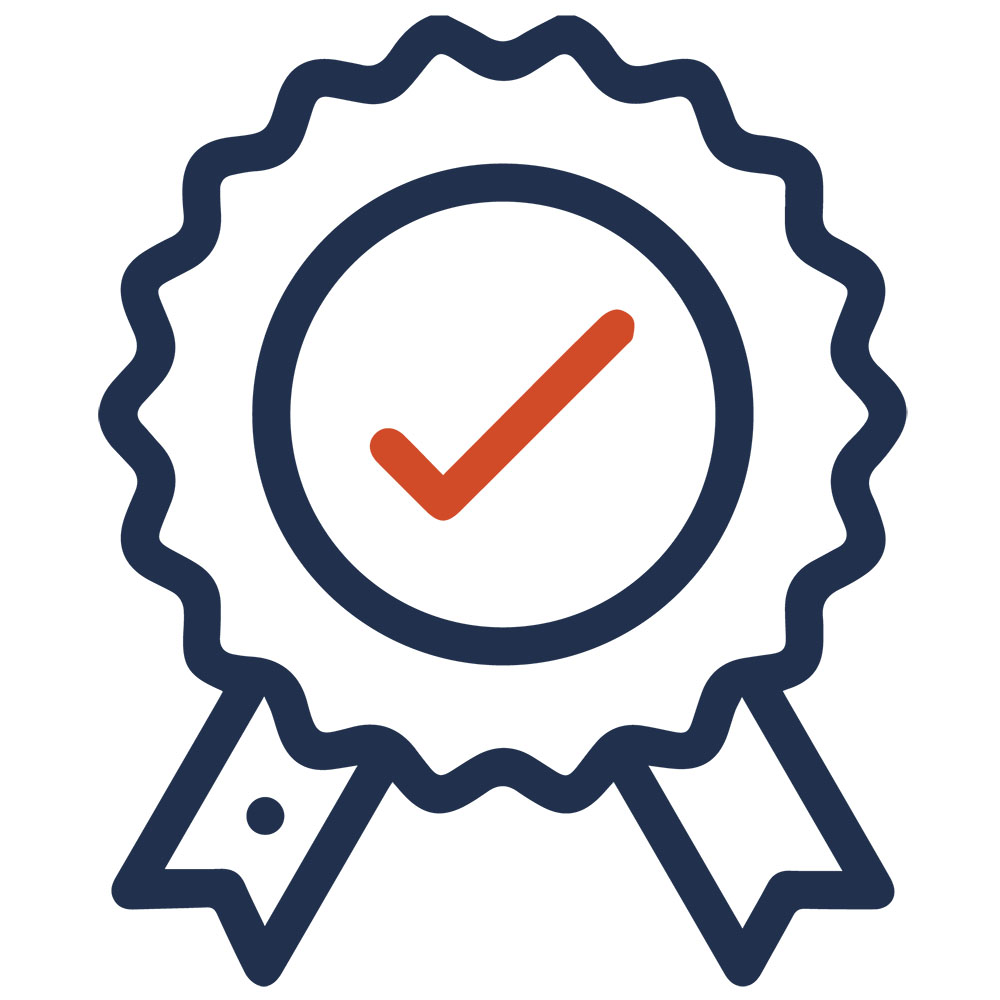
On average, 96% of graduates have positive outcomes following graduation, such as securing a job or advancing to graduate school
Visit Mines
IN PERSON OR VIRTUAL TOURS DAILY
Take a tour of our beautiful campus in Golden, Colorado, nestled in the foothills of the Rocky Mountains.
Rocking the headlines: the latest news at Mines

Beck Venture Center opens on Colorado School of Mines campus

Ice-cored hills in the Arctic could help us detect water on other planets
Mines researchers partner with NASA to survey pingos in Canada’s Northwest Territories.

Colorado School of Mines, Chevron announce partnership for Global Energy Future Initiative
Chevron to sponsor research and participate in working groups to tackle complex problems related to the energy transition.
Students in the news
Student stories offer a glimpse into the lives of the bright, ambitious individuals who attend Colorado School of Mines. These personal accounts showcase the unique experiences and perspectives of Mines students as they pursue their academic and professional goals.

Expand your network

941 organizations posted 3,360 jobs in Diggernet from 2021-2022
Future-proof your career
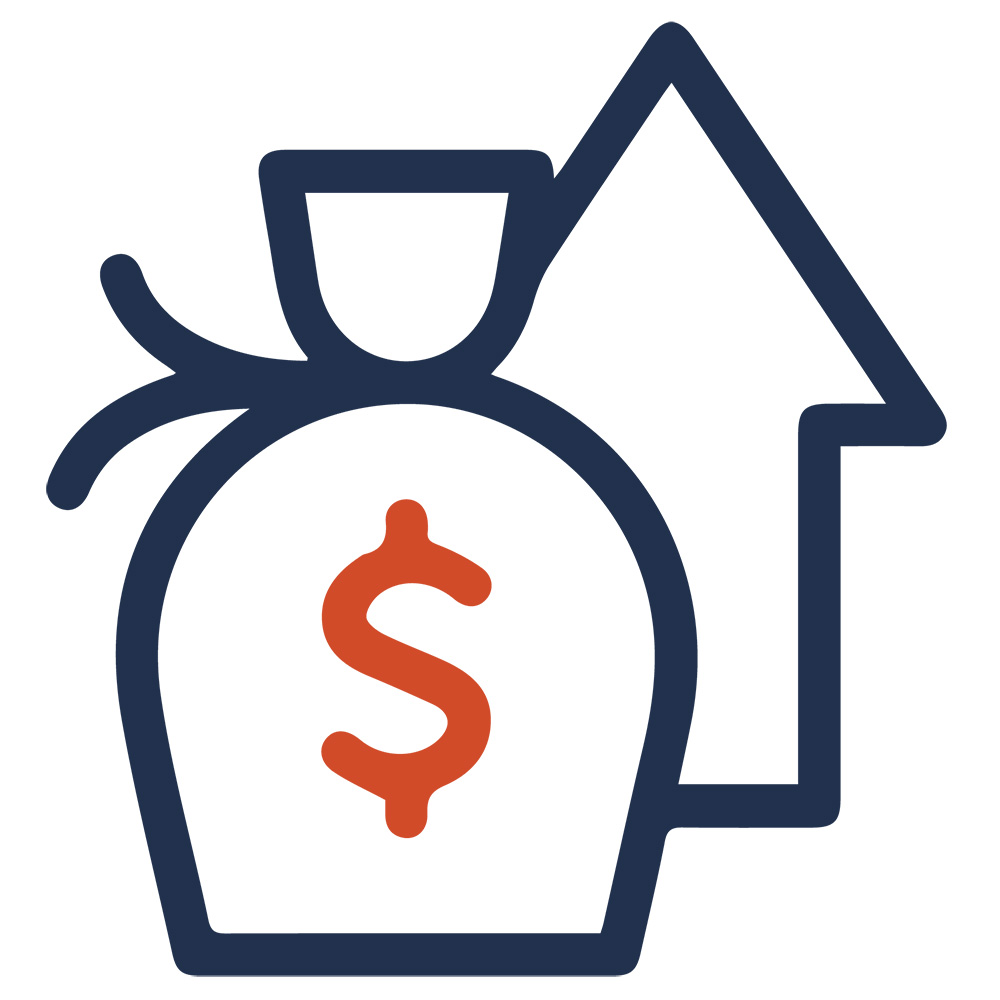
Top ranked best return on investment (ROI) Payscale, Georgetown University
Advance your education
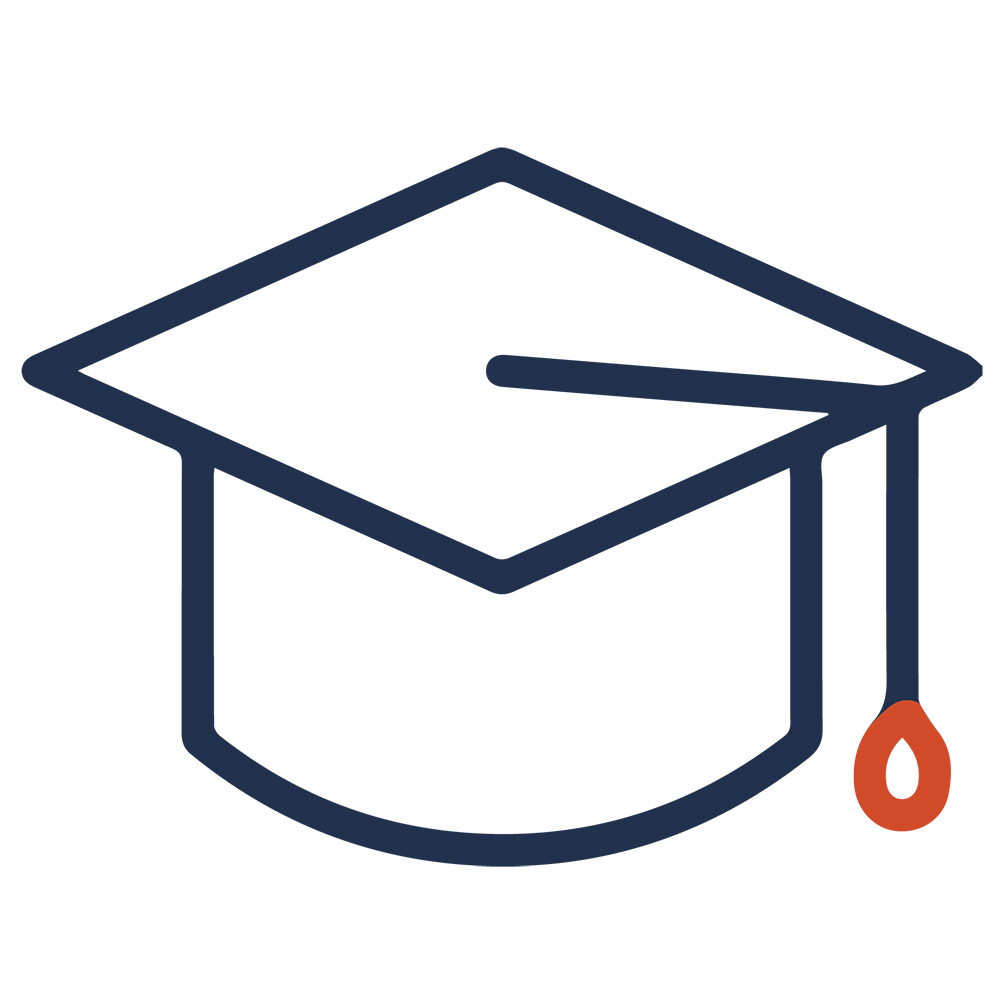
19% of Mines graduates continue with advanced education
Mines: The College Tour
Meet some of Mines’ students and an inspiring professor and learn about our university. Discover more about our exceptional academics and faculty, welcoming location and campus life, global focus, entrepreneurial and innovative mindset, unparalleled industry connections and numerous opportunities to change the world for the better.